Comparative Physiology and Biomechanics
I study the relationship between form and function by examining morphology and physiology at levels of organization from molecular to tissue or organ, and makes connections to whole-organism performance in the context of environment and evolution. Specifically, I use natural variation, experimental manipulations, and modeling, with the tools of muscle physiology and biomechanics, to study:
1) properties of components of musculoskeletal systems
2) integrated function of these components resulting in movement performance
3) changes in response to physiological or external perturbations.
Musculoskeletal systems are used for many extraordinary behaviors critical to survival and reproduction. Muscle is the source of energy in many movements and muscle contractile properties and the underlying physiological mechanisms are a continuing focus of cutting-edge research. However, movement also involves skeletal elements and other connective tissues, with complexity at levels of organization from molecular to tissue or organ. The integrated function of these components determines movement performance, so the properties of each component must be tuned to one another to achieve high performance. Movement must also occur under variable physiological and external conditions, and understanding how performance will be affected requires knowing how the properties of each component respond to perturbation and how this impacts the tuned, integrated function of the system.
Latch-Mediated, Spring-Actuated Movement
My research has focused on the role of elastic energy in animal movements. Rather than use muscle to move structures directly, in some animals muscles contract to stretch elastic structures storing energy while a latch prevents motion. After the latch is removed, the recoiling elastic structures release energy into motion of a projectile, either the body or an appendage. Movement performance achieved using elastic recoil is often much greater than what could be achieved using muscle alone in a similar context.
I have worked as part of a US Army Research Office Multidisciplinary University Research Initiative team of biologists, engineers, materials scientists, and physicists to understand the fundamental principles behind latch-mediated, spring-actuated movements. We recently published a commentary in the Journal of Experimental Biology describing the phenomenon and a framework for studying these systems.

From Longo, S. J., Cox, S. M., Azizi, E., Ilton, M., Olberding, J. P., St. Pierre, R. and Patek, S. N. (2019) Journal of Experimental Biology

Actuating motion using elastic recoil shifts the power demands of movement from muscle to a spring or elastic tissue. This bypasses the limits of muscle power output and any affects of temperature or other perturbations on that power. However, temperature can still impact elastically powered movements if it affects the total energy, or work, done during muscle contraction. Using in vitro contractions of frog muscle, we found that the work done during isotonic muscle contractions decreases at lower temperatures because of the decreases in muscle force at those temperatures. Additionally, the strength of temperature effects on muscle contracile velocity, power, and work depend on the force with which a muscle is contracting. Therefore, the way muscle contraction is affected by temperature and may impact movement performance depends on the way in which that muscle is being used.
Thermally Robust Movement Performance
Temperature is an external factor that impacts all aspects of physiology including movement performance using musculoskeletal systems. Movement performance tends to decrease at lower temperatures, in part because of the effects of decreasing temperature on muscle contraction. Elastically powered movements, like tongue projection in some salamanders, are less sensitive to changes in temperature compared with movements that are powered directly by muscle. We measured the contractile properties of salamander tongues muscle at different temperatures and found muscles used for elastically powered tongue projection respond to changes in temperature just as strongly as muscle from species without elastically powered tongue projection (Olberding et al., 2018). Insensitivity to temperature in this behavior may result from the use of elastic energy if recoiling elastic structures are not as strongly affected by temperature as muscle contraction.

From Olberding, J. P. and Deban, S. M. (2017) Journal of Experimental Biology
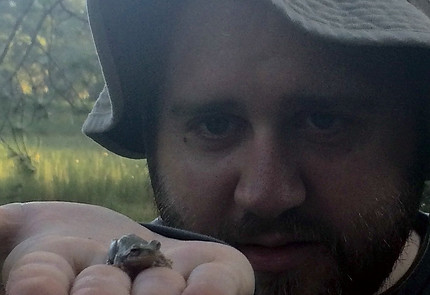
Size and Allometry
Size has dramatic consequences on organismal form and function, including movement using musculoskeletal systems. Because muscle contractile properties are important determinants of movement performance, understanding how these properties scale is key to predicting the scaling of whole-animal movement. Interestingly, jump performance is predicted to remain the same for animals of different size, assuming geometric similarity. Using in vitro experiments on frog muscle from and intraspecific range of body mass from 1.28 to 20.60 g, we found that the scaling of muscle work supports the prediction of constant jump performance at all sizes (Olberding and Deban, 2018).
Jumping performance is predicted to remain constant because both the energy required to jump and the energy available from limb muscle are proportional to body mass. However, small animals must push off from the ground in a much shorter time than larger animals, because of their shorter limbs. This places higher power (rate of energy transfer) demands on the muscle of small animals. Because muscle power is physiologically limited, small animals may actually have lower jump performance than larger animals. But if a small animal uses elastic recoil, they can avoid these power limits.
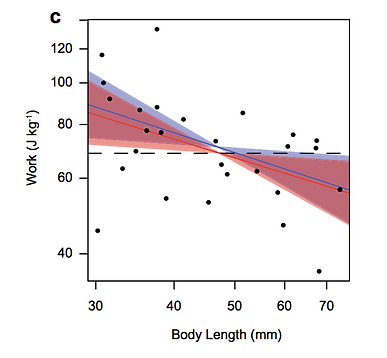
From Olberding, J. P. and Deban, S. M. (2018) J Comp Phys B
Although elastic recoil is useful for small jumping animals, it could be detrimental for jump performance in larger animals. The amount of energy that can be stored in a spring is defined by the relationship between the force-length properties of the spring and muscle. Only a portion of the potential work that can be done by a muscle can be stored in elastic elements and recovered into motion. So there must be some size at which muscle contraction without elastic elements does more work and results in greater jump performance than muscle contractions that store energy in elastic tissues. Data collected from studies of jumping animals support the conclusion that larger, muscle-actuated species have greater jump performance (Sutton et al., 2019)
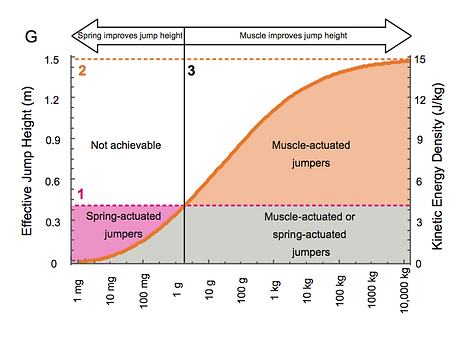
From Sutton, G. P., Mendoza, E., Azizi, E., Longo, S. J., Olberding, J. P., Ilton, M. and Patek, S. N. (in press) Integrative and Comparative Biology
Latching and Limb Morphology
In frogs, the latch that prevents spring motion during loading and allows spring motion during recoil arises, in part, from the dynamic mechanical advantage of the extending hind limb. Poor mechanical advantage at the start of the jump prevents motion while elastic elements are loaded, then increasing mechanical advantage as the joints extend allows for recoil. A pattern of increasing mechanical advantage may occur in any animal that jumps with extending limbs, regardless of the presence or properties of any elastic elements. By modeling this system, we found that increasing mechanical advantage creates disadvantageous conditions for muscle power generation without elastic elements. Therefore, the addition of elastic elements greatly improves power and energy output. Additionally, the morphology of the limb segments, combined with the mass being moved, determines the characteristics of the emergent latch and system performance. Finally, this system allows for muscle to do additional work during spring recoil, changing predictions for both spring properties and size-based transitions from spring actuation to direct muscle actuation.
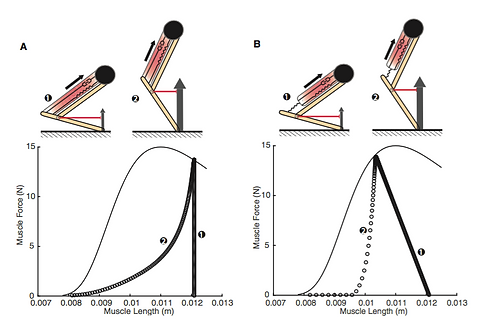
Undergraduate Mentees
In 2016, I mentored Marina Schwartz on her project comparing swimming and jumping performance in aquatic and arboreal frogs. She presented this work as a poster at SICB 2017 in New Orleans. She has since completed a M.S in
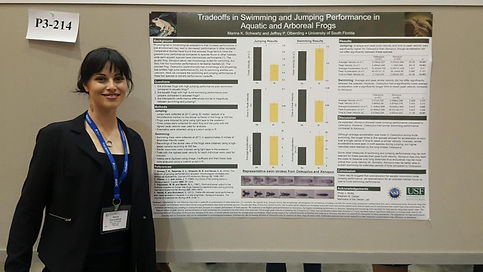
Other Projects
I earned my MS in Evolution, Ecology, and Organismal Biology at the University of California, Riverside while working with Dr. Tim Higham. My thesis focused on the jumping abilities of collared lizards (Crotaphytus bicinctores). A previous project examined the effects of obstacles in the environment on the locomotion of bipedal lizards (Aspidoscelis sexlineata).
I completed my senior honors thesis working in Dr. Bruce Jayne's lab. There I studied perception, behavior, and functional morphology in snakes. Using boa constrictors, I investigated the exploratory behavior of snakes in a nocturnal arboreal habitat to better understand how these animals function within their natural environment.
As an undergraduate I worked with Dr. Ken Petren to study the population genetics of invasive species. I helped to collect and process genetic samples from a population of introduced Italian Wall Lizards (Podarcis muralis) in the Greater Cincinnati area. I also assisted with the collection of genetic samples from invasive Tropical House Gecko (Hemidactylus mabouia) from Florida to assess patterns of invasion. Finally, I helped to determine the sexes of critically endangered Mangrove Finches (Camarhynchus heliobates) using gel electrophoresis to assist with conservation efforts in the Galapagos.